Department News
CHASE Solar Hub pioneers liquid fuel conversion
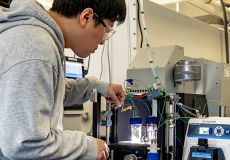
At the center's Chapel Hill headquarters, more than 100 researchers work to turn sunlight into methanol.
Inspired by Female Role Models, Julianne Hall Puts Her Stamp on Chemistry Education
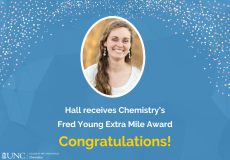
Julianne Hall wins Chemistry's Fred Young Extra Mile Award
Cookies with Professors doing Cool Research
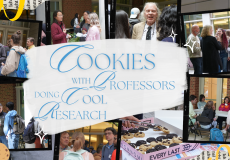
Photos of Chemistry undergraduate students and faculty eating cookies and talking.
Carolina undergraduate named 2024 Barry Goldwater Scholar
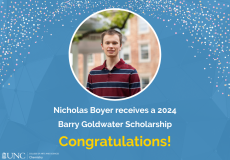
Nicholas Boyer, a rising senior at UNC-Chapel Hill double majoring in chemistry and computer science was selected to receive a 2024 Barry Goldwater Scholarship.
Research
Real-Time TDDFT for Nonequilibrium Electron Dynamics
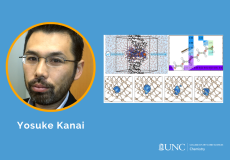
In the invited Perspective article for the Journal of the American Chemical Society, members of the Kanai research group discuss...
Monolayer-like Exciton Recombination Dynamics of Multilayer MoSe2 Observed by Pump-Probe Microscopy
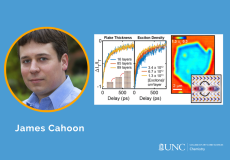
Transition metal dichalcogenides (TMDCs) have garnered considerable interest over the past decade as a class of semiconducting layered materials.