UNC Chemists Unveil Blueprint for Molecular-Scale Computers
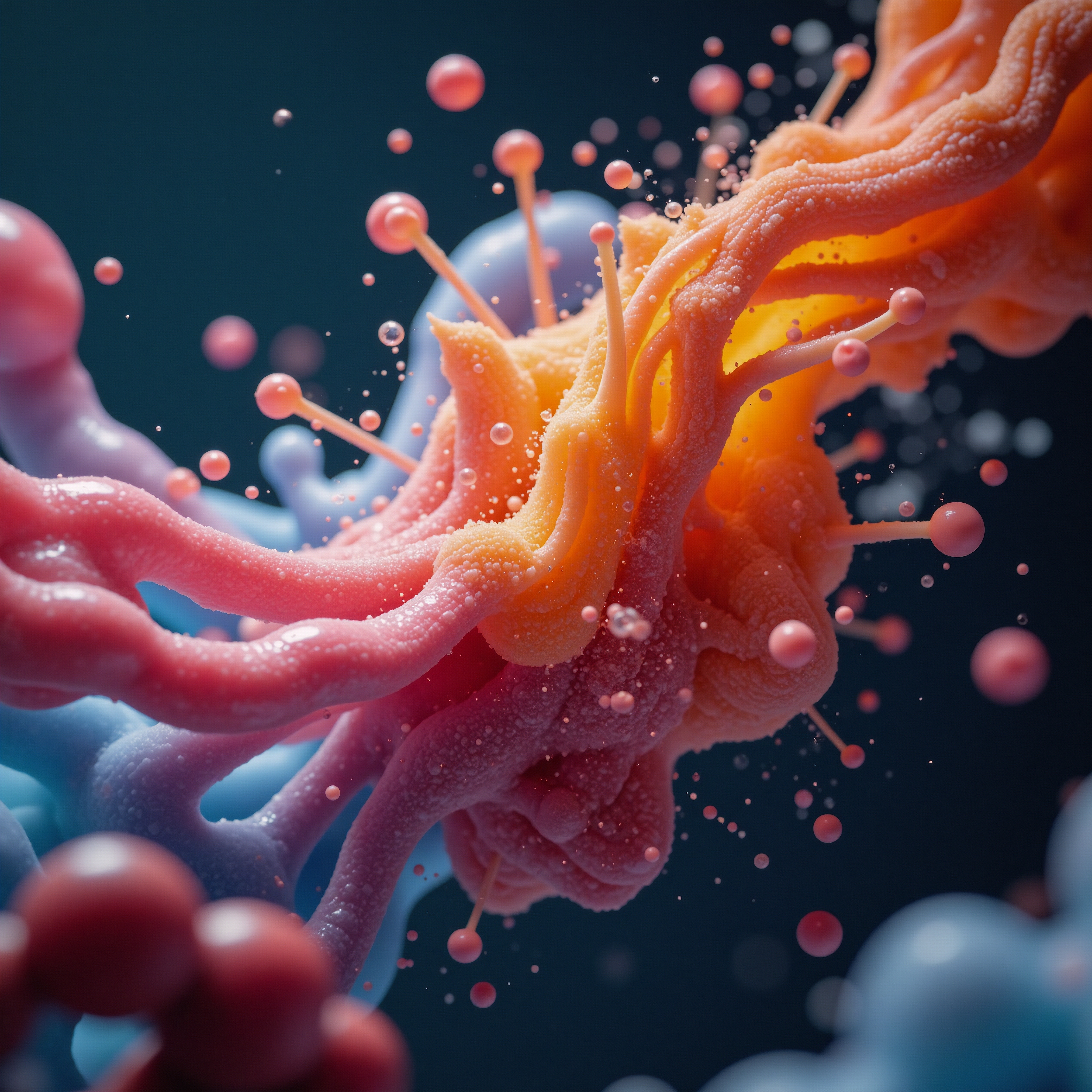
Instead of relying on microchips and circuits, the research of Dr. Zhiyue Lu, an assistant professor in the UNC Department of Chemistry, and Dr. Zhongmin Zhang, a postdoctoral scholar, explores how a single molecule—changing shape in response to its environment—can recognize patterns and process information.
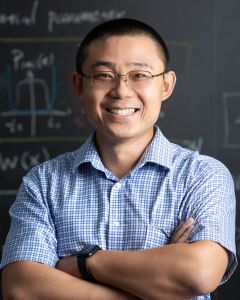
April 1, 2025 I By Dave DeFusco
For centuries, humans have built machines to process information—first with gears and levers, then with electronic circuits, and now, as scientists at UNC-Chapel Hill are discovering, with single molecules.
Dr. Zhiyue Lu, an assistant professor in the UNC Department of Chemistry, and Dr. Zhongmin Zhang, a postdoctoral scholar, are rethinking how computation can work at the smallest scale possible. Instead of relying on microchips and circuits, their research explores how a single molecule—changing shape in response to its environment—can recognize patterns and process information.
Their study, “Kinetic Frustration Enables Single-Molecule Computation,” published in the Journal of Chemical Physics, introduces a novel principle: thermal-kinetic frustration, which allows a molecule to behave like a tiny, programmable computer. This discovery not only advances the field of molecular computing but also opens doors to new applications in drug delivery, biochemical sensing and self-assembling materials.
At its core, computing is about recognizing and responding to patterns. Traditional computers process sequences of data—like text, numbers or images—by changing their internal states according to predefined rules. This process allows computers to store information, make decisions and solve problems.
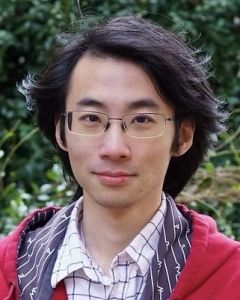
But what if a molecule could do the same thing? The UNC researchers propose that molecules can process information through a change in configuration in response to environmental signals, such as temperature changes, mechanical forces or chemical concentrations. These environmental conditions act like input signals, guiding the molecule along different physical pathways—similar to how a computer follows a set of instructions.
“Imagine a molecule as a tiny machine with multiple possible shapes or configurations,” said Professor Lu, who is senior author of the NSF-funded study. “As the environment changes over time, the molecule moves through a series of shapes, eventually settling into a final form that encodes the history of those environmental changes—just like how a computer processes a sequence of inputs to reach a final decision.”
To explain how molecules can perform computations, Lu and Zhang introduce a concept called the Energy Seascape. In traditional physics, molecules exist within an energy landscape—a metaphorical map showing all the possible shapes a molecule can take, with valleys representing stable states and hills representing energy barriers. But when a molecule is exposed to changing environmental conditions, its energy landscape is no longer fixed—it moves like waves in the ocean, shifting in response to temperature, force or chemical changes.
“This ‘dancing’ energy landscape forces the molecule to continuously adapt, preventing it from settling into equilibrium too quickly,” said Dr. Zhang, the lead author of the paper. “As a result, the molecule must navigate a sequence of temporary states before reaching a final shape, allowing it to store and process information in the process.”
One of the biggest challenges in molecular computing is thermal noise—the random motion of molecules due to heat. At such a small scale, thermal fluctuations can make it difficult for a molecule to remain in a specific state long enough to encode useful information.
To solve this, Lu and Zhang introduce the concept of thermal-kinetic frustration. This principle creates a built-in delay in how a molecule transitions between states, ensuring that it doesn’t immediately jump to the most stable configuration. Instead, the molecule must pass through a series of intermediate states, like a computer processing multiple steps before arriving at a final answer.
“This frustration acts as a control mechanism, allowing the molecule to follow a structured computational pathway rather than randomly fluctuating,” said Professor Lu. “It also enables the molecule to ‘remember’ the sequence of inputs it has received, much like a computer’s memory stores past information.”
To demonstrate their theory, the researchers designed a proof-of-principle single-molecule automaton, inspired by a concept in computer science called a finite state machine—a simple model of computation that processes sequences of inputs step by step. The molecular automaton is built from a polymer, a chain-like molecule made of smaller repeating units. Each unit can exist in one of two states: folded (compact) or unfolded (extended), much like a binary system of 1s and 0s.
By applying mechanical forces—stretching or compressing the polymer—the researchers can control which units fold or unfold, guiding the molecule through a structured sequence of configurations. The final shape of the polymer encodes the pattern of mechanical inputs it has received, demonstrating how a molecule can recognize and “compute” sequences of information.
While this study lays the theoretical foundation for single-molecule computing, the next step is real-world implementation. DNA, one of nature’s most sophisticated molecular machines, provides an ideal platform for testing these principles. DNA strands naturally fold and unfold based on their sequence, and scientists can design them to follow specific folding pathways.
“By leveraging DNA nanotechnology, we could build molecular computers capable of recognizing biochemical signals, detecting diseases or even triggering targeted drug delivery,” said Dr. Zhang.